Dual-Beam Laser Traps, Optical Stretcher, Optical Cell Rotator
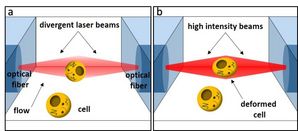
Optical trapping employs forces generated by light to spatially immobilize suspended objects in a contact-free manner. Arthur Ashkin was the first to demonstrate that microscopic objects can be trapped and manipulated with light. He went on to create a stable trap using two counter-propagating laser beams – the first dual-beam laser trap (DBLT) [1]. 16 years later, he also introduced optical tweezers, which utilize the forces of a single, focused laser beam [2]. In 1993, based on Ashkin’s early dual-beam work, Mara Prentiss and co-workers created the first DBLT employing two opposing optical fibers [3]. As the trapping optics of the fiber-based DBLT are completely separated from the microscope optics, DBLTs then became an extremely versatile tool for the micro-manipulation of biological samples. They are also easily combined with microfluidic lab-on-chip systems for efficient delivery of trapping objects.
Previous work in our lab [4] demonstrated that the optical forces in a dual-beam laser traps can be used to deform and measure soft materials in a controlled manner. When used for this purpose, DBLTs have come to be known as an optical stretcher (OS). An illustration of the trapping and deformation of a cell in the OS is shown in Figure 1. We have used the OS extensively to measure the viscoelastic properties of various cell types and have established cell deformability as a marker of cell function [5, 6, 7, 8, 9, 10, 11, 12]. Along the way we had improved the throughput of the OS to hundreds of cells per hour by combining it with microfluidic assemblies [13, 14]. With the advent of real-time deformability cytometry (RT-DC), with its 10,000x higher throughput, the OS has increasingly been repurposed for the high-content study of rare or sensitive objects, such as phospholipid vesicles [15] or isolated cell nuclei [16]. Previously, it had also been used to test the axial light transmission through individual cells, e.g. by gently trapping and aligning living Muller cells along their optical axis without any mechanical contact [17].
In current studies, we use DBLTs for the controlled rotation of single cells in an optical cell rotator (OCR) [18]. In the OCR, the output of one of the fibers, which is a few-mode fiber, is controlled by a spatial light modulator to achieve a rotating double-lobed mode profile (Figure 2). The optical forces induced by the rotating mode result in a rotation of the trapped cell. Rotating single cells about an axis perpendicular to the optical axis of a microscope allows the acquisition of tomographic data sets of individual cells. Besides the all-optical rotation in the OCR we also employ a combination of optical trapping in a DBLT with drag forces induced by flow in a microfluidic channel for a contact free optofluidic rotation of individual cells, as proposed by Kolb et al. [19]. We use these techniques together with QPI and ODT to determine 3D refractive index maps of individual cells [20].
All these examples showcase the versatility of DBLTs and propose it as a high-content tool for single cell studies.
[1] A. Ashkin, “Acceleration and trapping of particles by radiation pressure,” Physical Review Letters, vol. 24, p. 156–159, 1970.
[2] A. Ashkin, J. M. Dziedzic, J. E. Bjorkholm, and S. Chu, “Observation of a single-beam gradient force optical trap for dielectric particles,” Optics Letters, vol. 11, iss. 5, p. 288–290, 1986.
[3] A. Constable, J. Kim, J. Mervis, F. Zarinetchi, and M. Prentiss, “Demonstration of a fiber-optical light-force trap,” Optics Letters, vol. 18, iss. 21, p. 1867–1869, 1993.
[4] J. Guck, R. Ananthakrishnan, T. J. Moon, C. C. Cunningham, and J. Käs, “Optical deformability of soft biological dielectrics,” Physical Review Letters, vol. 84, iss. 23, p. 5451–5454, 2000.
[5] J. Guck, R. Ananthakrishnan, H. Mahmood, T. J. Moon, C. C. Cunningham, and J. Käs, “The optical stretcher: a novel laser tool to micromanipulate cells,” Biophysical Journal, vol. 81, iss. 2, p. 767–784, 2001.
[6] J. Guck, S. Schinkinger, B. Lincoln, F. Wottawah, S. Ebert, M. Romeyke, D. Lenz, H. M. Erickson, R. Ananthakrishnan, D. Mitchell, J. Käs, S. Ulvick, and C. Bilby, “Optical deformability as an inherent cell marker for testing malignant transformation and metastatic competence,” Biophysical Journal, vol. 88, iss. 5, p. 3689–3698, 2005.
[7] T. W. Remmerbach, F. Wottawah, J. Dietrich, B. Lincoln, C. Wittekind, and J. Guck, “Oral cancer diagnosis by mechanical phenotyping,” Cancer Research, vol. 69, iss. 5, p. 1728–1732, 2009.
[8] F. Lautenschläger, S. Paschke, S. Schinkinger, A. Bruel, M. Beil, and J. Guck, “The regulatory role of cell mechanics for migration of differentiating myeloid cells,” Proceedings of the National Academy of Sciences, vol. 106, iss. 37, p. 15696–15701, 2009.
[9] A. E. Ekpenyong, G. Whyte, K. Chalut, S. Pagliara, F. Lautenschläger, C. Fiddler, S. Paschke, U. F. Keyser, E. R. Chilvers, and J. Guck, “Viscoelastic properties of differentiating blood cells are fate-and function-dependent,” PLoS One, vol. 7, iss. 9, p. e45237, 2012.
[10] H. K. Matthews, U. Delabre, J. L. Rohn, J. Guck, P. Kunda, and B. Baum, “Changes in ect2 localization couple actomyosin-dependent cell shape changes to mitotic progression,” Developmental cell, vol. 23, iss. 2, p. 371–383, 2012.
[11] S. M. Man, A. Ekpenyong, P. Tourlomousis, S. Achouri, E. Cammarota, K. Hughes, A. Rizzo, G. Ng, J. A. Wright, P. Cicuta, J. R. Guck, and C. E. Bryant, “Actin polymerization as a key innate immune effector mechanism to controlSalmonellainfection,” Proceedings of the National Academy of Sciences, vol. 111, iss. 49, p. 17588–17593, 2014.
[12] C. J. Chan, A. E. Ekpenyong, S. Golfier, W. Li, K. J. Chalut, O. Otto, J. Elgeti, J. Guck, and F. Lautenschläger, “Myosin ii activity softens cells in suspension,” Biophysical journal, vol. 108, iss. 8, p. 1856–1869, 2015.
[13] B. Lincoln, H. M. Erickson, S. Schinkinger, F. Wottawah, D. Mitchell, S. Ulvick, C. Bilby, and J. Guck, “Deformability-based flow cytometry,” Cytometry Part A, vol. 59A, iss. 2, p. 203–209, 2004.
[14] C. Faigle, F. Lautenschläger, G. Whyte, P. Homewood, E. Martín-Badosa, and J. Guck, “A monolithic glass chip for active single-cell sorting based on mechanical phenotyping,” Lab on a Chip, vol. 15, iss. 5, p. 1267–1275, 2015.
[15] U. Delabre, K. Feld, E. Crespo, G. Whyte, C. Sykes, U. Seifert, and J. Guck, “Deformation of phospholipid vesicles in an optical stretcher,” Soft matter, vol. 11, iss. 30, p. 6075–6088, 2015.
[16] C. J. Chan, W. Li, G. Cojoc, and J. Guck, “Volume transitions of isolated cell nuclei induced by rapid temperature increase,” Biophysical Journal, vol. 112, iss. 6, p. 1063–1076, 2017.
[17] K. Franze, J. Grosche, S. N. Skatchkov, S. Schinkinger, C. Foja, D. Schild, O. Uckermann, K. Travis, A. Reichenbach, and J. Guck, “Müller cells are living optical fibers in the vertebrate retina,” Proceedings of the National Academy of Sciences, vol. 104, iss. 20, pp. 8287-8292, 2007.
[18] M. Kreysing, D. Ott, M. J. Schmidberger, O. Otto, M. Sch�rmann, E. Martín-Badosa, G. Whyte, and J. Guck, “Dynamic operation of optical fibres beyond the single-mode regime facilitates the orientation of biological cells,” Nature Communications, vol. 5, p. 5481, 2014.
[19] T. Kolb, S. Albert, M. Haug, and G. Whyte, “Dynamically reconfigurable fibre optical spanner,” Lab on a Chip, vol. 14, iss. 6, p. 1186–1190, 2014.
[20] P. Müller, M. Schürmann, C. J. Chan, and J. Guck, “Single-cell diffraction tomography with optofluidic rotation about a tilted axis,” Proc. SPIE, vol. 9548, p. 95480U-95480U-5, 2015.